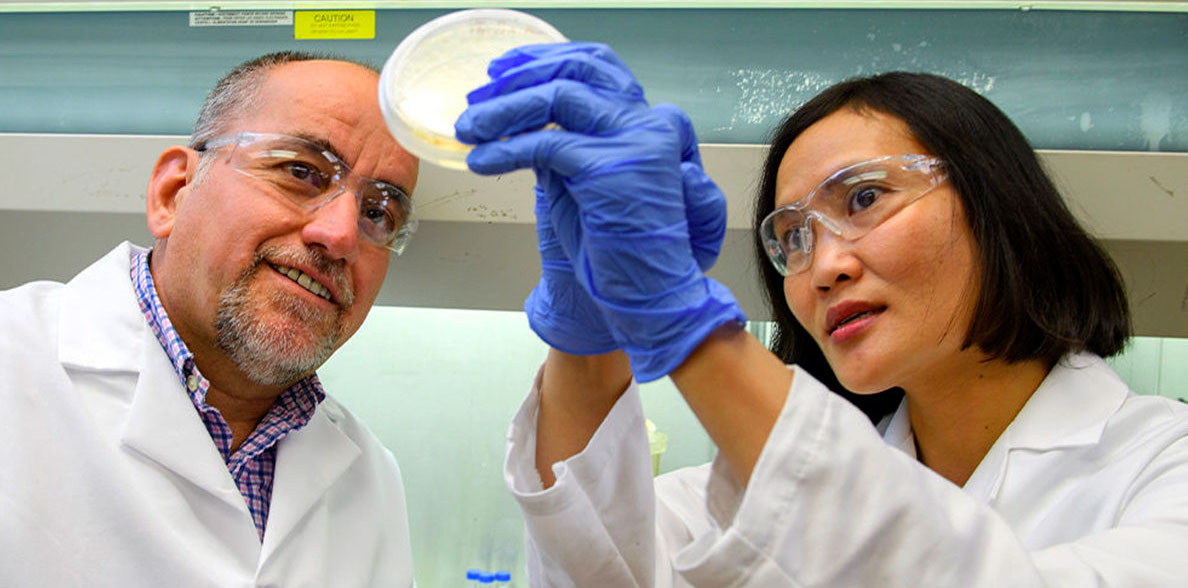
Research
Voiland College is working to power new research and solutions to create a sustainable energy future, to apply technology to preserve our quality of life, and to educate tomorrow’s innovators.
Research News
Contact Us
Questions about research? Contact the Voiland College Office of Research and Graduate Studies.